Biochar and hydrochar from sewage sludge (*WNWNW #3)
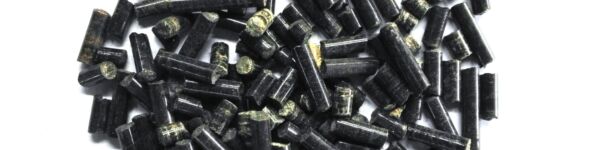
Simon Judd has over 35 years’ post-doctorate experience in all aspects of water and wastewater treatment technology, both in academic and industrial R&D. He has (co-)authored six book titles and over 200 peer-reviewed publications in water and wastewater treatment.
Waste Not Want Not Wednesdays #3: the relative benefits offered by the various thermochemical treatment technologies*
1. Sludge generation and end management
It is widely recognised that, despite being produced in much smaller quantities than the main sewage flow − less than 1% by volume if the raw sludge is assumed to contain ~2−3% dry solids − the cost of processing sludge is about the same as treating the main sewage flow (Domini et al, 2022). Traditionally, sewage sludge has been managed through landfilling and land spreading. Incineration is also widely employed as end treatment to significantly reduce the volume of material for end disposal (usually to landfill). However, increasingly stringent environmental regulations associated with concerns about the impact of micropollutants (heavy metals, pathogens, microplastics and dissolved organic contaminants − including PFAS), has prompted the development of alternative approaches for its end management (or disposal).
2. Thermochemical processes
Thermochemical processes (Fig. 1) employ elevated temperatures, some in combination with elevated pressures, to reduce/destroy the sludge solids content while also recovering latent energy and/or capturing some value from the organic carbon. Pyrolysis and hydrothermal carbonisation (HTC) form part of the suite of processes that apply heat and pressure, to varying extents, under oxygen-depleted conditions to convert the carbon component of the biomass into some sort of useful product(s) (Table 1). Pyrolysis and HTC are to be distinguished from oxidative thermochemical processes (sometimes termed ‘thermal processes’) that mineralise the carbon ostensibly to carbon dioxide, allowing the generated heat to be recovered. Of the oxidative processes, only incineration has been widely implemented for end treatment of sewage sludge. However, pyrolysis and HTC, which respectively form biochar and hydrochar as a carbon-rich solid residue, offer potentially more sustainable alternatives to incineration.
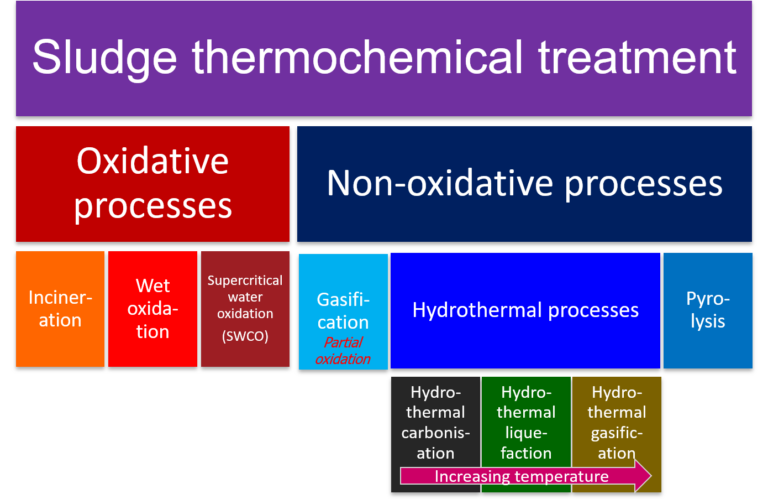
Technology | Output | Key advantage | Key challenge | Temperature °C, pressure |
---|---|---|---|---|
Incineration | Heat, electricity | 95−99% solids mineralisation | High emissions. Dry sludge feed | 850−1250, atmos. pressure |
Pyrolysis | Biochar, bio-oil/syngas | 50−70% solids conversion to useful solid/liquid products | Dry sludge feed | 300−700 |
Gasification | Syngas (CO, H₂) | 80−95% solids conversion; produces hydrogen | High temperature | 800−1200 |
Hydrothermal carbonisation (HTC) | Hydrochar | 40−60% solids conversion to useful products; dewatered sludge feed | Limited commercial use | 180−250, 20−40 bar |
Hydrothermal liquefaction (HTL) | Bio-crude oil | − | High pressure | 250−350, 100−300 bar |
3. Biochar vs hydrochar
Biochar and hydrochar share similar properties and applications (energy generation, soil amendment, carbon sequestration, and as adsorbents for gas and water/wastewater treatment), but their production methods and physicochemical characteristics differ. The pyrolysis process, which produces biochar, is a high-temperature process that requires a substantially dry feedstock (Table 1). HTC, which generates hydrochar, is a lower-temperature process requiring the presence of moisture. It can therefore be conducted on dewatered sludge without thermal drying.
Pyrolysis can be configured to produce liquid and gaseous products (bio-oil and syngas respectively) by adjusting the temperature profile. This also influences the biochar properties: higher temperatures typically increase its carbon content while reducing the yield. At the same time, the yield of bio-oil and/or syngas, which are also useful products, is increased. All inorganic matter, including phosphorus and metals, is retained in the biochar. The retention of phosphorus means that biochar can potentially be used in agriculture. However:
- this demands that the toxic metals are retained in the biochar matrix when employed for soil amendment, and
- the phosphorus must be in a bioavailable form − which excludes the ferric and aluminium salts generated by chemical phosphorus removal (CPR).
So, the use of biochar for soil amendment − an attractive option since it effectively sequesters carbon dioxide while also avoiding costly landfill disposal − relies on upstream implementation of biological phosphorus removal (BPR). If BPR is not in place, then there are both economic and logistical/spatial implications with BPR implementation.
HTC is a hydrothermal process (HTP) that operates at relatively low temperatures and moderate pressures (Table 1) and with a feedstock containing 75−90% moisture, such that thickened or, more usually, dewatered sludge can be used. This obviates the relatively high-energy thermal drying pre-treatment step. However, the process consequently generates an aqueous waste stream that requires further treatment, specifically to remove the phosphate and soluble heavy metals.
The partitioning of the inorganics into the aqueous phase, along with the lower production temperatures, mean that hydrochar has a higher carbon content than biochar. Biochar retains these inorganic materials and has a lower volatile carbon content at the higher production temperatures employed. Hydrochar is therefore a slightly better fuel than biochar, particularly when used directly in biomass boilers.
Finally, both biochar and hydrochar have other potential uses apart from for energy generation and soil amendment. Biochar can potentially be used as an adsorbent in anaerobic digestion biogas upgrading (Cavali et al, 2022), as well as for enhancing AD itself (Cavali et al, 2022; Capone et al, 2024). It has also been demonstrated that biochar prepared at elevated temperatures can effectively retain PFAS when used for soil amendment (Meservey et al, 2024; Sørmo et al, 2024), and that hydrochar can be used for toxic metals removal (Park et al, 2023). Biochar has also been assessed as a building material supplement (Onuaguluchi et al, 2024; Yang et al, 2024).
4. Life cycle analyses
The recent increased interest in thermochemical methods for end treatment of sewage sludge amongst the research community has been dramatic. Over the past five years, more than 40 life cycle assessments (LCAs), and a smattering of economic analyses, in this subject area have been published in the peer-reviewed literature. Such studies have generally – but not always – indicated the implementation of either pyrolysis or HTPs to provide a modest environmental benefit compared with land application. However, the outcomes are highly sensitive to the assumed boundary conditions and options.
Crucially, not all of those studies – around a dozen-or-so – that have included incineration in the analysis have concluded that pyrolysis and HTPs always offer a lower environmental impact. At least three assessments (Faragò et al, 2022; Havukainen et al, 2022; Yu et al, 2023) appear to suggest that incineration provides the lowest impact, under the specific conditions of each of these studies. Three other studies (Mannarino et al, 2022; Li et al, 2023; Gievers et al, 2025) concluded HTPs to offer a lower impact than (a) pyrolysis or incineration (Li et al, 2023; Gievers et al, 2025) or (b) composting (Mannarino et al, 2022), though Gievers et al (2025) also found pyrolysis to yield lower emissions than HTC when the biochar was used for soil amendment. Two further studies (Chang et al, 2025; Gievers et al, 2021) concluded pyrolysis to have a lower carbon footprint or global warming potential than incineration.
One analysis (Rydgård et al, 2024) has even concluded that direct use in agriculture of the unprocessed sludge represented the most sustainable option. This was mainly because the higher nutrient content in the form of nitrogen and bio-available phosphorus yielded greater plant growth, though this clearly must overlook the potential toxicity of the sludge due to its micropollutant content.
5. Conclusions
A cursory examination of the literature suggests that there is no single sludge processing route that consistently offers the lowest environmental impact under all circumstances. Ultimately the most favoured option is likely to depend on the sludge quality, upstream assets and processes (and specifically whether BPR has been implemented), sludge volumes and logistics. These are all site-specific – and may explain the differing outcomes of the many recent LCA studies published in this area. This is a classic case of exercising caution when presented with 'the new paradigm' – at least until it's clear that the old paradigm isn’t going to cut it.